Although the first orbital mission was eventually deemed a success for the payload, the Ascension Mk2 failed in its attempt to place the satellite it carried into a stable orbit. This brought the Ascension team back to the drawing board to improve the Viklun upper stage and make it more capable of maintaining attitude with its Reaction Control System cold gas thrusters. In the months since the first mission additional design work was also carried out for the next payload, dubbed Kerbin II and meant to test out technologies that would be used in the first line of communication satellites we are hoping to deploy in 2021. Once the Viklun stage improvements and payload design were finalized the rocket was assembled without issue in the VAB but the day before launch a problem occurred with installing the Radioisotope Thermal-electric Generator. The RTG was installed late in the launch preparation because the heat it outputs would compromise the fairings if left too long without thermal control, such as that provided by the crew access tower when the rocket is on the pad. Thankfully the problem was easy to overcome and the mission was only delayed to the following week.
The Flight
With no issues popping up during pre-flight the Mk2’s K2-X main engine lit up on time at T-3s under command of the AFCS, throttled at 10% power to check for good ignition. At T-0 after chamber pressures were confirmed to be nominal the 4 solid rocket boosters were ignited and their hold down bolts blown away to allow the rocket to begin its ascent with a combined force of 191kN to produce just over 1.6Gs acceleration. Immediately after launch all four of the rocket’s fins actuated to roll the rocket from 45° to 60° heading over about 6s before beginning to pitch over downrange. At the time pitch-over began the two horizontal fins nullified their ability to react to roll inputs so later in the flight at supersonic speeds control flutter would be kept to a minimum to reduce vibrations on the payload. By L+10s the SRBs had begun to taper off their thrust and the main engine throttle started to properly increase in order to maintain a TWR of 1.5.
The ascent continued nominally, with the guidance computer switching pitch profiles smoothly, and at L+34s while passing through 3.2km the four SRBs expired. They were immediately detached from their decouplers to drop away from the rocket, which throttled up the main engine from 80% to full thrust of 181kN (out of an eventual 195kN) to continue on with 1.4Gs of force and climbing. Pitch control remained nominal. The boosters all safely fell just off shore from KSC and were not thrown back towards the runways or launch complex.
L+56s into the flight the rocket passed through maximum dynamic pressure with a force of 17.558kPa @ 8.044km while traveling nearly 400m/s (all speeds for this mission will be reported as orbital velocity). Two seconds later it broke the sound barrier and entered into supersonic flight. The transonic regime is where fin flutter (also known as roll shake) begins to become an issue and sure enough as the rocket passed through Mach 1.3 at L+1m8s the control surfaces began to oscillate. The fin flutter continued throughout the remainder of the main engine burn however the rocket was not shook violently by this and remained largely on course.
Apokee was pushed out of the atmosphere at L+1m51s as the rocket continued a nominal ascent up through 34.4km with a velocity exceeding 870m/s. As it continued to climb and the air thinned out the guidance fins became less and less able to maintain proper pitch control with the range of motion allowed, traveling to the full extend of their movement. The rocket gradually began to drift off its nominal ascent angle without being able to lower the nose fast enough.
Main engine cut-off (MECO) occurred shortly afterwards at L+2m12s with the rocket traveling in excess of 1.3km/s and the lower stage was immediately decoupled with six small solid rocket motors pulling it back and away from the Viklun upper stage. Within a second later the second stage engine was ignited (SES-1) at full thrust to continue the push upwards with 68kN of force at 1.5Gs. The RCS ports were opened to allow the stage to maintain attitude and continue to pitch over. The thrusters were powerful enough to allow the stage to recover its pitch profile without over-compensating and losing control.
Passing through 60km at L+2m19s the payload fairings were confirmed to have been successfully detached, exposing the Kerbin II payload as the rocket continued upwards to escape the atmosphere altogether at L+2m28s. Viklun burn remained nominal and attitude remained nominal all the way up to the first second engine cut-off (SECO-1) at L+2m43s when the rocket’s apokee reached 210km. The off-nominal pitch prior to staging that allowed the rocket to climb more vertically brought SECO-1 earlier than expected, occurring almost 48s too soon but leaving the spacecraft with just over 1 ton of extra fuel.
8s after SECO-1 the RCS had re-oriented the rocket to face its prograde vector and the Stability Assist System (SAS) controlling the reaction wheels on Kerbin II had been activated to hold that orientation as the rocket continued up towards apokee. RCS was now disabled because it had used up way more fuel than expected, already draining its tanks down to nearly 50%. With just under 4 minutes to apokee controllers on the ground quickly worked to run the trajectory data through their faster computers to send orbital burn timings up to the rocket’s less-capable flight computer. The burn timings were uploaded at L+3m30s and controllers sat back to wait and hope the Viklun engine would re-ignite for orbital insertion burn.
OIB (SES-2) was successfully underway at L+6m10s with the Viklun engine at full thrust while the rocket continued to hold prograde orientation with its reaction wheels. The burn was set to last for a given amount of time, not dependent on factors like perikee height, so that we could properly test the results of our calculations on the ground. At L+6m58s perikee went positive and began to rapidly climb out of the atmosphere to 190km by the time of SECO-2 at L+7m5s. The Viklun stage, not just the payload, was now in a stable orbit for a successful launch! More than twice the amount of planned fuel remained for de-orbit later.
However mission success still hinged on proper deployment of Kerbin II, so celebration did not last long as controllers got back to work getting the satellite ready to be detached from the upper stage. First step was to purge the Viklun flight computer of instructions used for ascent and load it with instructions needed for on-orbit operations. Before this could be done the rocket passed out of view from DSN Central and we had to wait for it to be picked up again by Arekibo about 9min later – the only communications gap along its trajectory.
After the flight computer was properly setup the next step was to deploy the RTG away from the spacecraft so the heat it radiated would not fall so strongly upon it, especially since the rocket was coming back around into the sunlight. The extensible boom was successfully pushed out at L+38min and for additional thermal control the rocket was placed into a slow roll to remain evenly heated by the sun. This completed the rocket’s first full orbit around Kerbin.
After some more system initialization and testing, both the primary and backup communication antennae were deployed by L+50min so they could be tested and ensure that once the probe was deployed we would still be able to talk to it. At the altitude of its orbit, the tertiary emergency antenna would not be able to reach the ground, although it could still talk to us through the Viklun stage’s antenna as long as it remained in orbit and powered as well. Both antennae checked out just fine.
Nearing full deployment readiness the Viklun upper stage RCS was re-activated to kill its roll and re-orient the stage into a radial-out position so it pointed away from the planet along its trajectory. Because of the low RCS fuel levels and without any time restrictions only a short burst was used to begin the stage’s motion, slooowwlly rotating it to the proper orientation over a period of just over one minute before another short burst was used to cancel out the momentum so the SAS could be brought back online and use the reaction wheels to hold orientation.
With the rocket now properly positioned for payload deployment the final activation sequence was carried out to switch on Kerbin II’s batteries, open its fuel valves and prime the cold gas engine, then run a quick test of all RCS thruster ports to confirm functionality. With all that successful the probe was pushed free of the payload truss at L+1h41m31s. 10s later Kerbin II’s flight computer woke up and we started to receive telemetry data to confirm a successful deployment!
Without the probe, the Viklun stage now relied on its own battery power to remain functional, which under normal circumstances would have only lasted it another few hours. To extend its stay in space and help us determine if any orbital decay exists one of its two flight computers was shut down completely and the second was put into hibernation. Doing this means the two can no longer talk to each other for cross-checks but after the ascent this ability has become less important. If one computer were to completely fail or act improperly we could still switch to the second but from this point forward only one could be operated at a time.
The flight computer woke up briefly early the following day at 0445 local time for a health check and seeing no issues controllers returned it to hibernation. Later in the day at 1627 local time the computer woke up once more and this time remained awake while controllers prepared for the final step of the mission: de-orbiting the stage.
Preparation began 5min prior to SES-3 with the RCS being used sparingly to slowly re-orient the stage to face retrograde so the engine thrust would brake and lower the trajectory into the atmosphere. Because the probe was no longer attached the stage now had no reaction wheels and had to rely entirely on its RCS to maintain proper orientation during the 14s burn and facing retrograde while on orbit means following a moving target – so there was concern that cold gas would run out before the burn completed and cause the rocket to drift far enough off retrograde its perikee would not be lowered enough to intercept the atmosphere.
With both battery power and cold gas quickly diminishing SES-3 occurred as planned at L+1d1h14m26s with the rocket not perfectly retrograde and still struggling to maintain orientation with dampened-down RCS thrust to save fuel. However upon SECO-3 when propellants were expired we were able to confirm that despite the off-nominal orientation the trajectory had successfully been altered enough to bring the stage back down towards the atmosphere. Enough battery power remained that we could have received telemetry all the way down to plasma blackout but some issue caused the flight computer to crash just as the stage entered the atmosphere. Still, it was enough to allow us to confirm re-entry over the waters southeast of Ockr as planned. During its time in space the Viklun upper stage orbited Kerbin 37 times and traveled a distance of nearly 200,000km.
Flight Analysis
Ascent Failure Preparation
We knew for this mission that the ability of the Viklun stage to maintain its attitude was not 100% guaranteed. Even though work had been done to ensure the improved RCS thrusters had the power to rotate the stage, we were still unable to fully model their use while under aerodynamic forces during ascent. This meant there was still a chance the rocket would tumble out of control similar to the first mission and so additional planning was undertaken to attempt to account for two types of ascent failures.
The first ascent failure would be if the Viklun upper stage was unable to maintain attitude and went into a tumble without also breaking apart from stress. The previous mission had the rocket continue to burn its main engine until target apokee was reached but this time we had the flight computer prepared to cut the engine and coast out of the atmosphere before attempting to regain attitude control. We made sure to model the ascent so that the lower stage would push the apokee well clear of the atmosphere to give us the opportunity to recover the upper stage if needed. We could then re-light the engine to either increase apokee or attempt an orbital insertion. Insertion would be to a low perikee just above the atmosphere so the stage could be easily de-orbited with a limited amount of fuel remaining while leaving the Kerbin II probe to raise its orbit after deployment. This was all confirmed to be possible in Launch Vehicle Designer.
The second ascent failure was in the event that the Viklun upper stage failed to maintain attitude and broke apart, or failed to make orbital insertion recovering from the above failure. Thankfully in the case of Kerbin II we had the option of safely returning the satellite at any time since it was equipped with a heat shield and parachutes. While this would have led to a mission failure for both vessels, we would at least be able to make another attempt before the end of the year.
Thankfully neither of these scenarios were required but we certainly do not consider the extra planning effort a waste of time.
Ascent Guidance
This was the first orbital mission to use an optimized trajectory in LVD, similar to what was done for a sub-orbital Ascension Mk1 flight earlier this year. This means that instead of coming up with a possible guidance profile we know the flight computer can fly and seeing how close that brings us to our intended trajectory, we had LVD work up an ascent profile targeting our intended orbit, then had to come up with a guidance profile to match it. In the past these guidance profiles were based on a singular quadratic fit function that would compute the proper pitch angle for the entire ascent. With phasing we are breaking up the pitch profile to use multiple quadratic fit functions over the course of the ascent. This was the first time we used so many, breaking the ascent down into 9 phases.
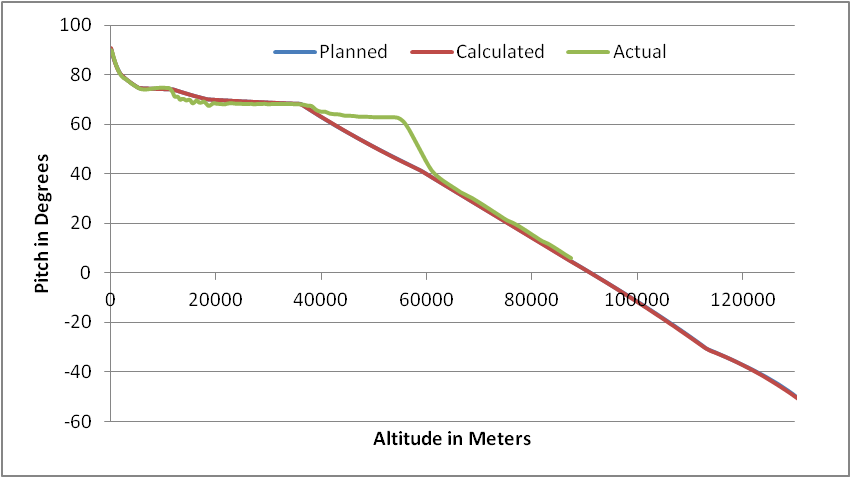
The Planned/Calculated plot ends when SECO-1 was supposed to occur. The Actual plot ends when SECO-1 actually occurred. Click for 4k version with additional annotation
The good news is that you can barely make out the planned plot under the calculated plot, which means the quadratic fit functions should have properly guided the rocket just as planned in LVD. This of course does not happen due to two reasons. First, the actual ascent deviates from planned when the rocket reaches Mach 1.3 and begins to suffer from fin flutter, making it impossible for the rocket to hold a precise orientation. As the fluttering dies out the path of the rocket again becomes closer to where it should be until the large pitch-over required just before 40km ASL. By now, despite our hopes, the air has grown too thin for the rocket’s guidance fins to maintain such a large change in pitch, they reach maximum deflection and the pitch angle flattens out. It gets better once the lower stage is dropped and the upper stage can use its RCS to properly orient and get back on track.
Although the actual track of the upper stage returns close to the planned/calculated track it still lags behind by 1-2 degrees. Why is this? Well it’s actually something we haven’t really noticed before thanks to lack of precise control later in flights due to fin flutter from control surfaces. With RCS able to maintain a steady pitch-over rate and with the pitch-over rate being higher than early in the flight we can see this discrepancy for the first time. What’s happening is the flight computer is telling the RCS where the rocket’s pitch should be at the current altitude, but the rocket doesn’t know this until the flight computer tells it, which means by the time its told what pitch angle to aim for it’s already passed that mark. This is why it is lagging just a degree or two behind where it should be. The rate of pitch is proper but the angle it’s supposed to be at is given too late for it to reach it on time.
An oversight that thankfully had no effect on the ascent was that we had not planned for SECO-1 to occur prior to the final guidance phase, which meant that after cutoff during phase 8 and pointing prograde, the guidance computer still attempted to bring the pitch onto the planned profile when the vessel reached the altitude for the final phase 9. It was not able to override the SAS however so the rocket remained pointing prograde and did not expend any extra cold gas attempting to change its pitch.
Ascent Discrepancies vs. Planning
In addition to guidance issues there were two other reasons why the rocket did not end up where we expected it to at SECO-1.
Despite strides made in improving the atmospheric simulation in LVD there appears to still be some work to do as the rocket’s actual performance does not equal what was planned, reaching a lower maximum dynamic pressure due to not accelerating as fast as planned. This could be also affected by the simulation’s handling of the SRB’s thrust curves and maintaining the proper TWR during the early phase of ascent (to be precise, the rocket’s TWR was slightly under 1.5 at 1.49 for the majority of the booster burn). The fact that the planned plot smoothly rejoins the actual plot however means it’s more likely linked to atmospheric modeling.
This discrepancy is more of our own doing, since although we used data from the first Ascension Mk1 mission it was not very fine grained and was also averaged over a wide range of altitudes. In the months since we have improved our ability to capture this parameter while in flight and you can plainly see where the first stage is let go at 54km and the fairings are detached at 60km. Considering this is the regime where the drag coefficient is highest the large discrepancy likely had a significant impact on our planning.
Orbital Insertion Burn Accuracy
Although the length of the burn and the timing of when the burn started should have put us into a nearly circular orbit, the one thing that was not accounted for was the burn vector, which was actually slightly off prograde The Mk2 flight computer was not able to handle this part of the maneuver which is why it ended up in a more elliptical orbit.
Orbital Decay
The reason we left the Viklun upper stage on orbit as long as possible was so that we could see if its trajectory was affected at all by the exosphere, which is a theorized part of the extreme upper atmosphere that extends past 70km into low-Kerbin orbit. We have not yet launched a spacecraft with instruments sensitive enough to detect it directly so if we saw the Viklun’s orbit affected by drag that would be a strong indication for the exosphere’s existence. With the Viklun stage being so much larger than Kerbin II it was a better candidate to make this detection but despite the extra time spent in orbit we were not able to definitively prove or disprove the exosphere’s existence. While the stage’s orbit did experience changes they were not large enough to be outside the error margins of our own ability to calculate the stage’s trajectory.
Future Plans
The Ascension Mk2 will remain active for now as an available design for reaching LKO and if the Mk3 development stalls or hits a setback over the coming months it could begin to place communications satellites into orbit in 2021. However the goal is to officially retire the Mk2 in favor of the Mk3, which should have its debut flight in Q1 2021. This was likely the final mission of the Mk2.
Along with improvements elsewhere the Mk3 will come with an upgraded guidance computer that should be able to better handle phased ascents in order to compensate for the lag and make sure the rocket’s pitch is as it should be when it should be. It should also be able to handle maneuver vectors so orbital insertions can be more accurate.
We will continue to work with the LVD author to improve its simulation and allow us to better model ascents to get more precise insertions into orbit. This will be integral in saving fuel for our upcoming Extremis mission in late 2021.
Although Kerbin II is much smaller than the Viklun stage it will remain on orbit a lot longer and we can potentially use it as well to determine of the exosphere exists and exerts drag on spacecraft in LKO.
Design tweaks to the Ascension Mk3 based on the performance of this flight are already underway and nearing final approval. We expect to have a final design to share by the end of the month.